Characterising the Atmosphere of Proxima B
✅ Paper Type: Free Essay | ✅ Subject: Physics |
✅ Wordcount: 2061 words | ✅ Published: 11 Sep 2017 |
1.Intro
As of April 2016, 582 extrasolar planets have been discovered (NASA) using Doppler spectroscopy, a method for detecting extrasolar planets by deducing their radial velocity from the Doppler shifts in the spectrum of the planets parent star. Using this method, Anglada-Escude et al (2016) recent observations have revealed a 1.3 Earth mass planet orbiting the red dwarf Proxima Centauri.
Although Earth-like planets aren’t uncommon, the recent discovery of Proxima b is particularly exciting as at a distance of 1.3 parsecs it is our closest potentially habitable star. Where previous studies were stunted by the distance of the host star to the Earth, Proxima b may within reach of Earth and space based telescopes giving us the ability to better judge its characteristics.
While Proxima b is in the goldilocks zone, meaning it has potential to have water and perhaps even life, it is subject to intense radiation and solar wind. ‘The habitability of Proxima Centauri b‘ and ‘Prospects for characterising the atmosphere of Proxima Centauri b’ question whether Proxima has an atmosphere, with the primary focus being whether Proxima b can maintain liquid water on its surface. The former does this by estimating the current high-energy irradiance of the planet and show that the orbit is likely to be in either a synchronous or 3:2 spin-orbit resonance. The latter article considers measuring the variation in thermal emission with orbital phase as a method for characterizing Proxima b’s atmosphere.
2. The habitability of Proxima Centauri b
2.1 Summary
In this paper the authors work through a number of factors that could affect the potential habitability of Proxima b. While noting there is a surplus of Earth like planets (Kepler), the authors need question whether Proxima lies within the Habitable Zone, where a stellar flux is low enough to maintain surface water but high enough to maintain the planetary surface as defined by Kasting et al. Due to a stellar input of ~65% than of the Earth Proxima b lies within these limits.
High Energy Irradiation
High-energy emissions including X-rays through to far-UV (FUV) and particle winds are known to influence atmospheres of rocky planets. A particular issue when estimating the XUV fluxes is that Proxima is a flare star (haish et 1983) meaning its HE emissions can vary significantly.
The work in the paper was built from X-ray emission observations via ROSAT and XMM that report log LX = 27:2 erg sô€€€1 g and LX =
27:4 erg sô€€€1 respectively (Haish 1999). To generalise the overall dose on the planetary atmosphere, the authors consider the average XUV luminosity over an extended timescale while accounting for flare variation. Using XMM-Newton observations, the strongest flare has an energy of about 2×10^31 erg. CN Leo has a similar X-Ray luminosity and thus can be used to model flare distribution for Proxima. Audard el state a power law with the form N(> E) = 3:7 _ 1037Eô€€€1:2, where N is the number of flares per day, and E is the total (integrated) flare energy in erg . This shows CN Leo has flares with energies greater than about 2 _ 1031 erg over a timescale of 1 day.
This is found to be in agreement with the XMM-data set, consolidating this is a reliable representative of the daily flux. Thus giving (between 0.65 and 3.8nm) a time integrated average flux of 87 ergss_1cm^-2 . To account for more energetic flares a calculated extra 22 ergs s^-1 cm^-2 is added, totalling at 109 erg s^-1cm^-2 . Flux estimates (including flare contribution) were calculated over a range of wavelengths, as listed below:
- ROSAT observations for the 3.8nm to 10nm range produced an X-Ray dose of 163 erg s^1 cm^2
- EUVE spectrum for the extreme – UV 10nm to 40nm range produced a flux value of 111 ergs^1cm2
- FUSE observations for the far – UV 92-118nm range produced a flux value of 20 egs
- HST/STIS for the 118-170nm range produced a flux value of 130 ergs
Therefore between 0.6 and 118nm, the total integrated flux today that is a fair representative of HE radiation on the atmosphere of Proxima b is of 307ergs^1. For comparison purposes, the XUV flux at Earth is calculated to be 5.1 ergs, showing Proxima b to receive 60 times more XUV flux. This data is illustrated in fig.
-Probability of capture:
The authors remark on the poor parameterizations of previous work on tidal dissipation of rocky planets (Darwin 1880, love 1909, goldreich 1963), their primary issue being models would always predict an equilibrium rotation rate where tidal torque would vanish. The authors use the Andrade model as tailored by Efromsky 2012 and Eq 10 of Marakov 2012. The Andrade time was set to be equal to the Maxwell time of tm=500yr for simplicity. The results for 3:2 resonance capture probability are shown in fig.
Curve a) indicates where tidal torque at the lower boundary of the separatrix is negative and greater than the maximum restoring torque. b) indicates tidal torque at the lower boundary of the separatrix is positive and (c) indicates where maximal tidal torque inside the resonance is greater than the maximum triaxial torque. Regions above (c) and/or (b) (white regions) leads to certain capture due to tidal torque, whereas below (c) and (a) (black region) capture is impossible as triaxial torque is too weak.
The implication for climate would be that liquid water is possible depending on the rotation. If the planet’s orbital eccentricity is <0.06, this could result in synchronous rotation, with one blazing hot side permanently facing towards the star, while the opposite side is in permanent darkness and freezing cold. In the asynchronous case where eccentricity is > 0.06 it is likely Proxima will have a tropical belt.
-water loss
It is undeniable how estimates of volatile loss are poorly constrained, due to the lack of key data e.g. evolution of atmospheric composition, stellar wind properties, photochemistry of the upper atmosphere etc. The authors take a moment to discuss an alternative approach for determining Proxima b’s volatile loss: Comparing Earths early development with Proxima b’s. By considering how Earth built by a multitude of moon size protoplanets across 10Myr, the authors argue the XUV irradiation and stellar wind experience on proto-Earth is comparable to that of Proxima b. lamer et al modelled volatile losses of early Earth showing significant loss caused by hydrodynamic escape of hydrogen and strong stellar wind exposure. Due to the fact the runaway phase of Proxima is only longer than the Earths by a small factor <10, one may assume the losses experienced by Earth that did not inhibit habitability, may also not cause a threat to the habitability of Proxima b. 2.2 Implications/Importance
This paper is important because it is one of the most through and recent investigations of the atmosphere and characteristics of Proxima b, yielding interesting conclusions.
The results showing Proxima b receives 60 times more XUV radiation than current Earth, poses a big question mark over its habitability. However, authors show that if reasonable constraints are put on orbital history and past irradiation, Proxima b could currently have a dense atmosphere and perhaps only a marginal loss of volatiles. Also comparison to Earths early evolution is particularly relevant, seeing as despite experiencing strong solar emissions, Earth has maintained a dense atmosphere. Perhaps further investigation into similarities should be considered to determine Proxima b’s current and future state.
Although this is a recent paper, results are already being used in others. B references this paper and makes use of findings in modelling Proxima b’s internal structure, subsequently deriving the radius of the planet. The authors conclude that assuming that Proxima b is solid, its radius is in the ∼0.94-1.40 R⊕ range. It is encouraging to see Angladas work giving way to further characterisation of Proxima b.
Also in terms of future research, this paper encourages the work of Breakthrough Starshot, a research project working to develop a spacecraft designed to make the journey to the Alpha Centauri star system. This could include a trip by Proxima b if more research proved it capable of hosting life. While not immensely strong support for potential life, it non-the-less makes research similar to this more promising.
It’s worth noting the authors have a follow up paper (turbet) which attempts to simulate Proxima b’s atmosphere and water cycle using 3D Global Climate Model for 1:1 and 3:2 spin-orbit resonances. They conclude it will be possible to image Proxima b and potentially determine habitability within a decade by proposing that atmospheric characterization will be possible via direct imaging with forthcoming large telescopes and the James Webb Space Telescope could be used for observation of thermal phase curves.
2.3 Assessment
The paper in general is an enjoyable read, although heavily scattered with references, all information was explained thoroughly meaning background reading of referenced work wouldn’t be necessary. The paper had a logical structure and covered a range of factors that would help determine Proxima b’s atmosphere. It is evident an extensive amount of research has been done by the authors and they have tried to portray the level of uncertainty and limitations of characterising said atmosphere. For the most part, methodology was thoroughly explained, especially where estimations/approximation were used.
However, when estimating the flare distribution for Proxima based on audards work on CN Leo, the authors note that results in Walker 1982 and Kunkel 1973 suggest the Proxima has about 60% the flare rate of CN Leo. The explanation as to why this isn’t an issue for current calculations felt a little vague. There is also a considerable difference in the flux value for the far-uv range calculation in comparison to 3.8nm to 10nm, 10-40nm and 118-170nm, which is seemingly important but not explained.
Additionally, when discussing probability of capture in spin-orbit resonance, it is explained why the Andrade model is used but the model itself is not described nor is ‘Eq (10) of Makarov’, making it hard to appreciate the calculated probability values. However, the updated methodology for capture probability uses more realistic parameterizations and is one that can be replicated for further work on Proxima b or similar planets. The authors also note that oversimplified tidal models can predict ‘pseudosynchronous rotation’ and that is a possibility that remains to be investigated.
Finally, Table 1 summarises the fundamental characteristics of Proxima Centauri and Proxima b, such core values are used throughout the work. As can be seen, the vast majority of values are based on previous work by the same author of this paper (anglada). If an error in judgement was made in a previous work it could lead to errors in current and future work. Stellar and planetary characteristics of the Proxima system ought to be checked via other research.
3. PROSPECTS FOR CHARACTERIZING THE ATMOSPHERE OF PROXIMA CENTAURI b
3.1 Summary
In this paper, Kreidberg and Loeb debate whether the soon to be completed James Webb Space Telescope (JWST) can be used to detect Proxima b’s thermal emission and subsequently determine whether Proxima b has an atmosphere. For a tidally locked planet with an atmosphere, one would expect heat redistribution from dayside to nightside as the planet orbits.
The toy climate model:
This was used to predict the infra-red phase variation of Proxima b with assumption that Proxima b is tidally locked. For a planet of no atmosphere, they considered a zero redistribution case, i.e. the nightside receives no flux, for a planet with an atmosphere they took a moderate redistribution of F=0.35. Fig 2 show the results of using this model.
The peak to trough phase variation at 10um is 35 ppm for the ‘rock case’ compared to 17ppm for the atmosphere case.
This is to be expected, in the case of an atmosphere, heat is redistributed to the nightside and thus the amplitude would logically be scaled down by ~a factor of two.
The authors then simulated a measurement of the thermal phase variation for Proxima b based on the work of Slsis et al, defining the phase variation as the difference between the star and planet spectrum at phase 0.5 and phase 0.0. These calculations produce Fig 2.
The blue and red lines corresponding to zero redistribution and 35% redistribution respectively, using simulated MIRI imaging measurements >12 um.
Finally, to determine how tightly the albedo and heat redistribution can be constrained, an MCMC fit was trailed on the simulated LRS spectrum from Fig 2 using the emcee package (Foreman-Mackey et al) The fit was run with redistribution, F, and albedo, A, as free parameters, from the fit one can measure F=0.07 and albedo A=0.13 to be well constrained.
The authors conclude that a MIRI phase curve is sufficient means to determine the existence of an atmosphere on Proxima b.
A then turn their attention to the feasibility of detecting an ozone absorption feature from Proxima b at 10um. Key motivation being this is a prominent feature of Earths IR emission spectrum and acts a potential evidence of present life. Bond albedo was set to A=0.3 to match Earths’ and an isothermal temperature arrangement is assumed. Using a modelling calculated by Rugheimer, that assumes an Earth-like atmospheric composition irradiated by a GJ 1214b-like star, it is noted that the ozone feature does not vary with planet orbital phase but is detectable from a very high signal-to-noise spectrum. The predicted feature amplitude was less than 1ppm. To illustrate how much observation time would be required to detect said feature, fig shows a simulated spectrum co-added from 60 days. The absorption feature at 9.8um corresponds to an ozone band and the dip at 8 is due to methane.
3.2 Implication/Importance
A major concern would be that detecting modulations with JWST would be extremely challenging due to Proxima Centauri’ s stellar variability and flares. To estimate how feasible it would be to detect Proxima b’s thermal emission, the JWST Exposure Time Calculator (ETC) was used to estimate signal-to-noise ratios for MIRI observations of Proxima Cen. Using a model spectrum, the ETC produced the expected count rate, which also correlated with the S/N predictions from Cowan et al. Although it feeds back good results, the paper highlights the need to test the precision of the MIRI detector.
A general issue with this paper is calculations are simply based on possible observations with the James Webb Space Telescope, as opposed to actual recorded data. Realistically, conclusions drawn about phase variation for Proxima aren’t definitive, though the paper shows potential of the MIRI could do and show. Other recent paper including ….., have a general agreement that the JWST could be used to infer exoplanets rotation periods and dissipation rates. In theory, measurement using the MIRI (which covers the wavelength range of 5 to 28 microns) at different wavelengths could be used to find atmospheric signatures, constrain the radius, the albedo and the inclination of Proxima b, as well as its rotation. This paper consolidates this idea.
3.3 Assessment
In the introduction many issues that could affect Proxima b’s atmosphere are mentioned, for example Proxima b’s evolutionary history, but these topics weren’t covered in the body of the article. The introduction also states three methods available to characterize the planet’s atmosphere. The last (measure variation in thermal emission with orbital phase) is the focus of this paper, however the first two (directly image the planet, measure variations in reflected starlight with orbital phase) were perhaps unreasonably undiscussed.
The quality of the results is difficult to assess, on the one hand an abundance of assumptions were used, to the extent that all of Section Five of this paper lists assumptions about the planetary system used in the their analysis. In many cases decisions were poorly explained. For example an albedo of 0.1 is used in the climate model while commenting Proxima b’s atmosphere would likely have a higher albedo (perhaps 0. Like Earths), however don’t they elaborate on why they settled with 0.1. On the other hand many of the assumptions were heavily based on previous work by a variety of researchers. The need for atmosphere case with moderate distribution F=0.35 was driven by a plethora of research ((e.g., Joshi et al. 1997; Merlis & Schneider 2010; Heng et al. 2011a, 2011b; Pierrehumbert 2011; Selsis et al. 2011; Leconte et al. 2013; Yang et al. 2013, 2014; Koll & Abbot 2015, 2016; Turbet et al. 2016).) which have shown how the presence of an atmosphere can reduce the amplitude of IR phase variation by a factor two or more. This made decisions feel more reliable and justified.
Yet, without having read referenced work, certain aspects were hard to follow. Some of their most significant work on simulated spectrum is noted to be built upon the work of selsis et al, however there is no indication of what work Sel actually conducted. The paper in general could’ve done with a more substantial amount of explanation for this made for a frustrating read.
4. Comparison
In terms of writing style,
It is apparent anglada had done a more extensive amount of research,
Work is important as it highlight the value of using a combination of space and ground telescopes and
In terms of research, both papers take a focus on the effects of different eccentricities on a tidally locked planet and its atmosphere. K considered this when creating a climate model and simulated a measurement of the thermal phase variation whereas anglada deduced it is likely Proxima is in a 3:2 rotation and considered the implication of the HE radiation Proxima b would receive.
Generally, the authors draw the same conclusion, that Proxima b could have an atmosphere, perhaps capable of supporting life. In the case of no heat redistribution or having synchronous rotation, Proxima b having an atmosphere is improbable. In the case or heat redistribution or asynchronous rotation, one could infer that an atmosphere or ocean is present. There’s a consensus that defining planet habitability is not straightforward, there are vast limitations on calculations that can be done and any modelling is not strongly representative of Proxima b’s characteristics.
Both papers also make use of modelling Earths past and current state as a forecast for Proxima b’s development. Using the Earth’s past to give us more information to further determine Proxima b’s atmosphere and potential habitability was fairly clever and intuitive concept. Future work, perhaps after the launch of the JWST, could consider this concept in more detail for a more well-rounded understanding of Proxima b’s characteristics.
In conclusion, both papers were particularly insightful, encourage future missions similar to Kepler, and will hopefully drive new research.
Cite This Work
To export a reference to this article please select a referencing stye below:
Related Services
View allDMCA / Removal Request
If you are the original writer of this essay and no longer wish to have your work published on UKEssays.com then please click the following link to email our support team:
Request essay removalRelated Services
Our academic writing and marking services can help you!
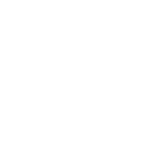
Freelance Writing Jobs
Looking for a flexible role?
Do you have a 2:1 degree or higher?
Study Resources
Free resources to assist you with your university studies!